The Benefits of a Lactobacillus to Your Health
Lactobacillus is a type of bacteria of which Lactobacillus gasseri is one type. You can find these ‘friendly’ Lactobacillus bacteria naturally in the human gut, urinary tract, and genital system. We can also get these helpful bacteria from certain foods and from dietary supplements called probiotics.
In general, Lactobacillus helps the body to break down food, absorb nutrients, and fight disease-causing organisms. Lactobacillus is also useful for preventing and treating diarrhea. Specifically, it is helpful for fighting diarrhea related to antibiotics use (1).
In this article, we’ll look more closely at probiotics and outline some health benefits of taking a Lactobacillus gasseri probiotic.

What are Probiotics?
Every human being is born with a certain ‘gut flora’. This flora consists of beneficial microorganisms, like bacteria, that you inherit for your mother. After birth, other helpful flora begins to ‘colonize’ your body. Although this may sound like some sort of alien invasion, it isn’t.
These beneficial or ‘friendly’ bacteria perform a number of roles in your body including immune system support, synthesizing certain vitamins, and converting fiber into acids (2).
So, probiotics are live microorganisms that are good for your health. Taking probiotics as a dietary supplement can help you boost your gut flora, the healthy bacteria in your gut (3). When taken in the correct amounts, your gut is healthier, and so are you!
Specific Health Benefits of Lactobacillus Gasseri
Lactobacillus gasseri is a probiotic which is useful for a number of health conditions. In addition to its general contribution to digestive and gut health, Lactobacillus gasseri probiotic is helpful for a number of other health conditions:
Weight Loss
According to some research, Lactobacillus gasseri couple possibly encourage weight loss and reduce abdominal fat. A British Journal of Nutrition study published in 2013 surveyed 210 obese adults.
These people were either given milk enriched with Lactobacillus gasseri or a placebo (milk without Lactobacillus gasseri). In the end, the Lactobacillus group saw an 8.5% reduction in abdominal fat compared to the placebo group after 12 weeks (4).
Vaginal Health
Lactobacillus gasseri probiotic also promotes vaginal health by preventing bacterial vaginosis. However, it is most effective when taken as a suppository. What’s more, studies suggest that Lactobacillus gasseri probiotic may also decrease menstrual pain in women (5).
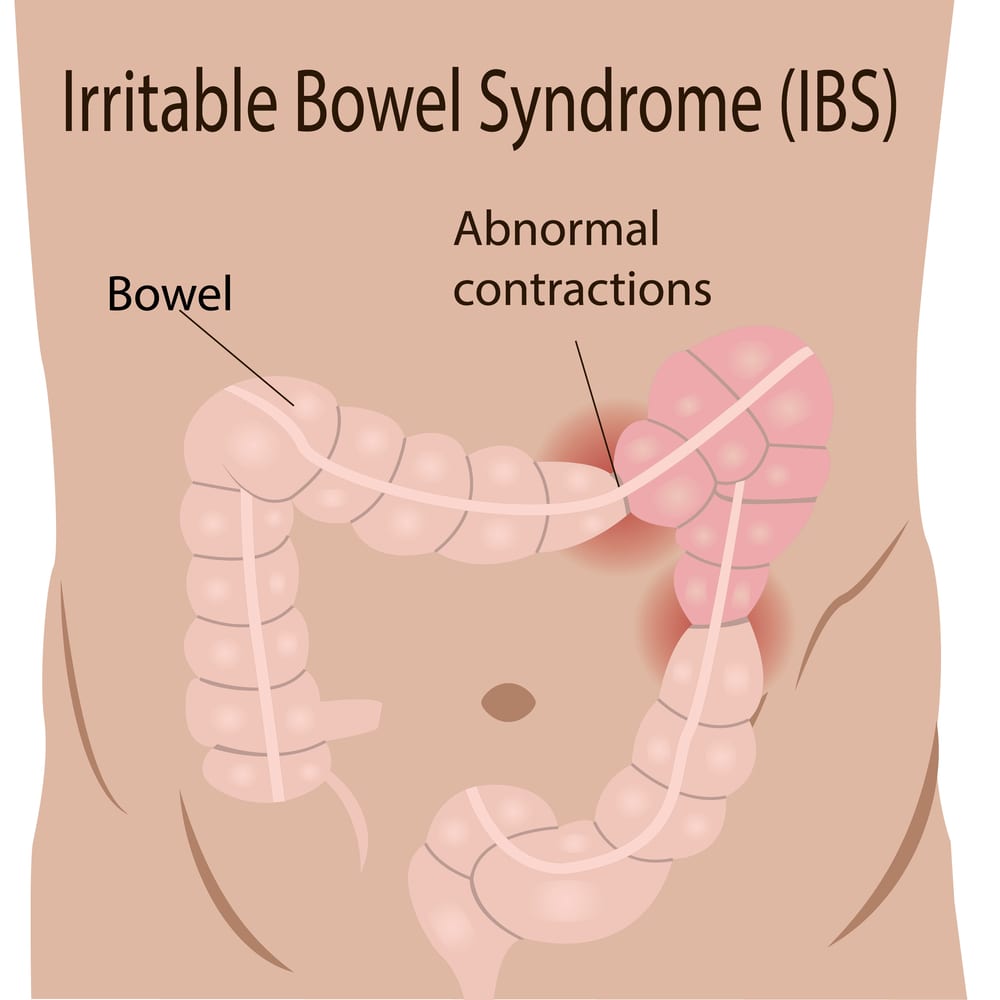
Cholesterol
In one study, participants received a product containing Lactobacillus gasseri and insulin. This product helped reduced total blood cholesterol, LDL (low-density cholesterol), lipoproteins, and triglycerides in both men and women with high cholesterol (8).
Boosts Immunity
Heat-killed Lactobacillus gasseri has been shown to boost immunity in elderly adults by increasing the number of T cells (9).
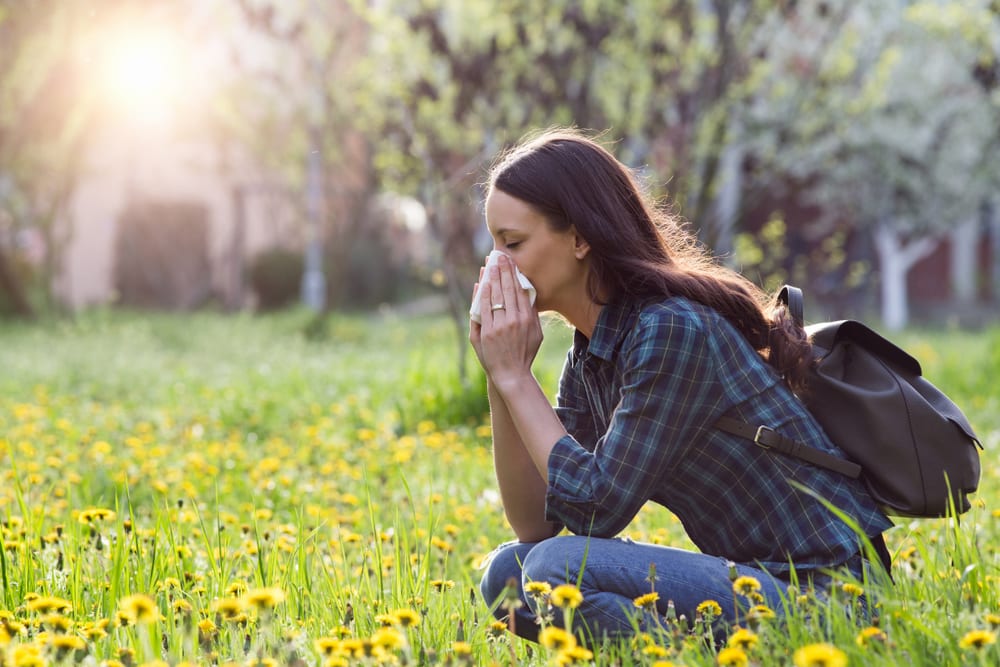
Allergies
In one study involved participants with an allergy to Japanese cedar, heat-killed Lactobacillus gasseri improved their nasal symptoms and enhanced their immune response (10).
Fatigue
When university athletes were given Lactobacillus gasseri probiotic after strenuous exercise, they experienced less resting fatigue. On top of that, Lactobacillus gasseri probiotic also elevated their moods (11).
Summary
Lactobacillus bacteria are friendly microorganisms that are native to the human gut. They help with a number of normal processes and help keep us healthy. Generally speaking, Lactobacillus helps you break down food, absorb nutrients, and fight certain unhealthy microorganisms. What’s more, it also supports your immune system support and helps your body synthesize certain vitamins. When our gut flora is out of balance, our health can suffer.
In addition to balancing gut flora, taking Lactobacillus gasseri probiotic is beneficial for a number of other reasons. It is frequently used for treating and preventing diarrhea, especially diarrhea caused by antibiotics. Furthermore, it boosts vaginal health in women and eases menstrual pain in women with endometriosis.
References
- https://www.webmd.com/vitamins/ai/ingredientmono-790/lactobacillus
- https://www.sciencedirect.com/science/article/pii/S0092867412001043
- https://www.webmd.com/drugs/2/drug-155565/probiotic-colon-support-oral/details
- https://www.ncbi.nlm.nih.gov/pubmed/23614897
- https://www.ncbi.nlm.nih.gov/pmc/articles/PMC3080472/
- https://www.verywellhealth.com/the-benefits-of-lactobacillus-gasseri-88697
- https://www.ncbi.nlm.nih.gov/pmc/articles/PMC3295086/
- https://www.ncbi.nlm.nih.gov/pubmed/20965319
- https://www.ncbi.nlm.nih.gov/pubmed/25653155
- https://www.ncbi.nlm.nih.gov/pubmed/19734682
- https://www.ncbi.nlm.nih.gov/pubmed/24195623
- https://selfhacked.com/blog/l-gasseri/
- source
9 Ways Lactobacillus Acidophilus Can Benefit Your Health
L. acidophilus is a beneficial bacteria found in your intestines that helps protect against various illnesses. To boost levels, consume fermented goods or take supplements.
Probiotics are becoming popular food supplements.
Interestingly, each probiotic can have different effects on your body.
Lactobacillus acidophilus is one of the most common types of probiotics and can be found in fermented foods, yogurt and supplements.
Lactobacillus acidophilus is a type of bacteria found in your intestines.
It’s a member of the Lactobacillus genus of bacteria, and it plays an important role in human health (1Trusted Source).
Its name gives an indication of what it produces — lactic acid. It does this by producing an enzyme called lactase. Lactase breaks down lactose, a sugar found in milk, into lactic acid.
Lactobacillus acidophilus is also sometimes referred to as L. acidophilus or simply acidophilus.
Lactobacilli, particularly L. acidophilus, are often used as probiotics.
The World Health Organization defines probiotics as “live micro-organisms which, when administered in adequate amounts, confer a health benefit on the host” (2Trusted Source).
Unfortunately, food manufacturers have overused the word “probiotic,” applying it to bacteria that haven’t been scientifically proven to have any specific health benefits.
This has led the European Food Safety Authority to ban the word “probiotic” on all foods in the EU.
L. acidophilus has been extensively studied as a probiotic, and evidence has shown that it may provide a number of health benefits. However, there are many different strains of L. acidophilus, and they can each have different effects on your body (3Trusted Source).
In addition to probiotic supplements, L. acidophilus can be found naturally in a number of fermented foods, including sauerkraut, miso and tempeh.
Also, it’s added to other foods like cheese and yogurt as a probiotic.
Below are 9 ways in which Lactobacillus acidophilus may benefit your health
High cholesterol levels may increase the risk of heart disease. This is especially true for “bad” LDL cholesterol.
Fortunately, studies suggest that certain probiotics can help reduce cholesterol levels and that L. acidophilus may be more effective than other types of probiotics (4Trusted Source, 5Trusted Source).
Some of these studies have examined probiotics on their own, while others have used milk drinks fermented by probiotics.
One study found that taking L. acidophilus and another probiotic for six weeks significantly lowered total and LDL cholesterol, but also “good” HDL cholesterol (6Trusted Source).
A similar six-week study found that L. acidophilus on its own had no effect (7Trusted Source).
However, there is evidence that combining L. acidophilus with prebiotics, or indigestible carbs that help good bacteria grow, can help increase HDL cholesterol and lower blood sugar.
This has been demonstrated in studies using probiotics and prebiotics, both as supplements and in fermented milk drinks (8Trusted Source).
Furthermore, a number of other studies have shown that yogurt supplemented with L. acidophilus helped reduce cholesterol levels by up to 7% more than ordinary yogurt (9Trusted Source, 10Trusted Source, 11Trusted Source, 12Trusted Source).
This suggests that L. acidophilus — not another ingredient in the yogurt — was responsible for the beneficial effect.
SUMMARY:L. acidophilus consumed on its own, in milk or yogurt or in combination with prebiotics may help lower cholesterol.
Diarrhea affects people for a number of reasons, including bacterial infections.
It can be dangerous if it lasts a long time, as it results in fluid loss and, in some cases, dehydration.
A number of studies have shown that probiotics like L. acidophilus may help prevent and reduce diarrhea that’s associated with various diseases (13Trusted Source).
Evidence on the ability of L. acidophilus to treat acute diarrhea in children is mixed. Some studies have shown a beneficial effect, while others have shown no effect (14Trusted Source, 15Trusted Source).
One meta-analysis involving more than 300 children found that L. acidophilus helped reduce diarrhea, but only in hospitalized children (16Trusted Source).
What’s more, when consumed in combination with another probiotic, L. acidophilus may help reduce diarrhea caused by radiotherapy in adult cancer patients (17Trusted Source).
Similarly, it may help reduce diarrhea associated with antibiotics and a common infection called Clostridium difficile, or C. diff (18Trusted Source).
Diarrhea is also common in people who travel to different countries and are exposed to new foods and environments.
A review of 12 studies found that probiotics are effective at preventing traveler’s diarrhea and that Lactobacillus acidophilus, in combination with another probiotic, was most effective at doing so (19Trusted Source).
SUMMARY:When consumed in combination with other probiotics, L. acidophilus may help prevent and treat diarrhea.
Irritable bowel syndrome (IBS) affects up to one in five people in certain countries. Its symptoms include abdominal pain, bloating and unusual bowel movements (20Trusted Source).
While little is known about the cause of IBS, some research suggests it might be caused by certain types of bacteria in the intestines (21Trusted Source).
Therefore, a number of studies have examined whether probiotics can help improve its symptoms.
In a study in 60 people with functional bowel disorders including IBS, taking a combination of L. acidophilus and another probiotic for one to two months improved bloating (22Trusted Source).
A similar study found that L. acidophilus alone also reduced abdominal pain in IBS patients (23Trusted Source).
On the other hand, a study that examined a mixture of L. acidophilus and other probiotics found that it had no effect IBS symptoms (24Trusted Source).
This might be explained by another study suggesting that taking a low dose of single-strain probiotics for a short duration may improve IBS symptoms the most.
Specifically, the study indicates that the best way to take probiotics for IBS is to use single-strain probiotics, rather than a mix, for less than eight weeks, as well as a dose of less than 10 billion colony-forming units (CFUs) per day (25Trusted Source).
However, it’s important to choose a probiotic supplement that has been scientifically proven to benefit IBS.
SUMMARY:L. acidophilus probiotics may improve symptoms of IBS, such as abdominal pain and bloating.
Vaginosis and vulvovaginal candidiasis are common types of vaginal infections.
There is good evidence that L. acidophilus can help treat and prevent such infections.
Lactobacilli are typically the most common bacteria in the vagina. They produce lactic acid, which prevents the growth of other harmful bacteria (26Trusted Source).
However, in cases of certain vaginal disorders, other species of bacteria begin to outnumber lactobacilli (27Trusted Source, 28Trusted Source).
A number of studies have found taking L. acidophilus as a probiotic supplement can prevent and treat vaginal infections by increasing lactobacilli in the vagina (29Trusted Source, 30Trusted Source).
Nevertheless, other studies have found no effect (31Trusted Source, 32Trusted Source).
Eating yogurt that contains L. acidophilus may also prevent vaginal infections. Yet, both of the studies that examined this were quite small and would need to be replicated on a larger scale before any conclusions could be made (33Trusted Source, 34Trusted Source).
SUMMARY:L. acidophilus as a probiotic supplement may be useful in preventing vaginal disorders, such as vaginosis and vulvovaginal candidiasis.
The bacteria in your intestines help control food digestion and a number of other bodily processes.
Therefore, they influence your weight.
There is some evidence that probiotics may help you lose weight, especially when multiple species are consumed together. However, the evidence on L. acidophilus alone is unclear (35Trusted Source).
A recent study that combined the results of 17 human studies and over 60 animal studies found that some lactobacilli species led to weight loss, while others may have contributed to weight gain (36Trusted Source).
It suggested that L. acidophilus was one of the species that led to weight gain. However, most of the studies were conducted in farm animals, not humans.
Furthermore, some of these older studies used probiotics that were originally thought to be L. acidophilus, but have since been identified as different species (37Trusted Source).
Therefore, the evidence on L. acidophilus affecting weight is unclear, and more rigorous studies are needed.
SUMMARY:Probiotics may be effective for weight loss, but more research is needed to determine whether L. acidophilus, in particular, has a significant effect on weight in humans.
Healthy bacteria like L. acidophilus can boost the immune system and thus help reduce the risk of viral infections.
In fact, some studies have suggested that probiotics may prevent and improve symptoms of the common cold (38Trusted Source, 39Trusted Source).
A few of these studies examined how effectively L. acidophilus treated colds in children.
In one study in 326 children, six months of daily L. acidophilus probiotics reduced fever by 53%, coughing by 41%, antibiotic use by 68% and days absent from school by 32% (40Trusted Source).
The same study found that combining L. acidophilus with another probiotic was even more effective (40Trusted Source).
A similar study on L. acidophilus and another probiotic also found similar positive results for reducing cold symptoms in children (41Trusted Source).
SUMMARY:L. acidophilus on its own and in combination with other probiotics may reduce cold symptoms, especially in children.
How To: Culture Lactobacillus (LAB) for Horticultural use
Generally when it comes to bacteria and microbes we’d be referring to the aerobic type you’d hope to produce in a Compost Tea (AACT) system, the reason being that the presence of anaerobic bacteria in these systems are nearly always ‘bad news’. However there are useful anaerobes out there and it is very much worth looking in to putting them to use in your horticultural endeavours!!
Enter Lactobacillus….
Lactobacillus is a facultive anaerobe that we are generally interested in for it’s ability to ferment a wide variety of things. It is this process that makes Lactobacillus or LAB the cornerstone of a range of processes the savvy gardener will find extremely useful. I’ll mention more about that later in this piece and in further blogs, but lets show you how to culture your own Lactobacillus first….
Step 1 – Rice wash
Technically you can use any reasonable carbohydrate source (preferably not simple sugars) but in this instance we’ll go with a Rice wash – I will be trying other more exciting things in the future, but until then….. Well the title says it all really, wash some rice and collect the water. This milky wash will now contain some of the starches from the rice and provide a food source for your bacteria.
Step 2 – Collect your initial culture
Place your rice wash in a suitable vessel (a jar…) and protect the neck with some kind of net to stop anything random getting in. Ideally you’ll want to place this outside, in a garden, on a balcony ect away from the elements but open to the air. This will allow the bacteria to go to work on the wash. A day or so should be fine. You will notice a change in the wash as the bacteria start to work, it will start to smell slightly sour and three distinct layers should be visible. You now need to collect the middle of these layers – the best way is with a siphon, but a syringe or whatever you have to hand will work – just try not to disrupt the layers.
Step 3 – Feed the LAB
Now it’s time to culture just the LAB that are present and nothing else. To do this we add milk to the liquid we collected at about 10:1, so for every 10ml of liquid you want to add 100ml of milk – You can use pretty much any milk as it’s the LAB in the wash we are culturing, however the least adulterated milk you can get your hands on the better. It’s probably worth saying you can’t use a lactose free milk for fairly obvious reasons….Finally we want to store this in an anaerobic state, so you have a few options – Ideally you can use a container with an airlock – the same as homebrewers use (or make one), you could use a bottle or jar and release the pressure every so often (not the best plan) or as I have use a heavy lid with a seal so any gas can escape but will then re-seal (not ideal to be honest….go buy some airlocks, you’ll want them for further projects!)
Step 4 – Prep & Store the LAB
After about a week you should notice a distinct change – You’ll have a layer of curds and a liquid layer – whey. It’s this liquid layer we want. Nothing too stressful here, just use a sieve and collect the liquid in a vessel – The curds can be put on the compost or whatever, it will be a great addition. Again your brew should smell sour (actually quite pleasant if you’re in to sour beers at all….) but not rancid, if it is bin it. OK, now you have your liquid you have 2 options, store it in the fridge where it will keep for about a week or mix it with Molasses to stabilise the culture where it will keep for 6 months or more. To stabilise mix the culture 1:1 with molasses, so 1 litre culture to 1 Litre of Molasses gives you 2 Litres…..it’s worth airlocking this too until the mix stabilises.
What’s the point?
Excellent question :o) The more mundane uses for LAB include using it as an odour neutraliser if you happen to keep chickens etc – Mix 30ml per litre of water and spray around the coop to reduce the smell – Unblock drains – 15ml per litre and let it go to work over night and many more! For your growing needs however mix 30ml or so with every litre of your plant’s water. The microbes will help cycle the nutrients in the soil making them more available to the plant! Add your LAB to compost – 30ml per litre and damp down every time you add to the pile or as you’re layering up. The Lactobacillus will speed up decomposition and start to cycle the nutrients! Finally (and more excitingly), I mentioned earlier that LAB is the cornerstone of further processes that are highly beneficial to a gardener. For instance LAB can be used for Bokashi composting, no more need to buy bran for your indoor composting! If you’ve never heard of Bokashi, I’ll cover it at some point. LAB can also be used to ferment plant material, for instance if you already add seaweed meal to your feeding regime, imagine if you could ‘pre-digest’ the nutrients held within the seaweed – making the non soluble elements readily available at application….with LAB you can. If you’re a gardener familiar with the process of rotting comfrey or nettles in a bucket to annoy your plot mates, why not use LAB to break down the vegetable matter without the smell, and more importantly, without the risk of culturing the bad anaerobic bacteria. Using these principles it’s basically possible to make your own organic liquid plant food for free and without losing friends or neighbours….. The last point for this post is probably my favourite – With LAB it’s possible to create your own fish fertiliser (Fish hydrolysate) this in conjunction with your nettle/seaweed/comfrey/grass brews will give you the perfect base for making your own liquid organic fertiliser…. …that’s not bad for a little milk and help from a bacterium.
Foot notes – There should really be a sequence of pictures to go with this post, but frankly they weren’t up to scratch. If anything needs clearing up drop me an email or comment below. – N.D source
LACTIC ACID BACTERIA: GARDEN AND SOIL BENEFITS(click Here)
Lactobacillus spp. for Gastrointestinal Health: Current and Future Perspectives
Abstract
In recent decades, probiotic bacteria have become increasingly popular as a result of mounting scientific evidence to indicate their beneficial role in modulating human health. Although there is strong evidence associating various Lactobacillus probiotics to various health benefits, further research is needed, in particular to determine the various mechanisms by which probiotics may exert these effects and indeed to gauge inter-individual value one can expect from consuming these products. One must take into consideration the differences in individual and combination strains, and conditions which create difficulty in making direct comparisons. The aim of this paper is to review the current understanding of the means by which Lactobacillus species stand to benefit our gastrointestinal health.
Introduction
Ilya Ilyich Mechnikov (Elie Metchnikoff), a Nobel Laureate for his work on macrophage phagocytosis, is credited as the first to propose that the gut microbiota could be manipulated to benefit the host. Mechnikov believed that putrefactive activity of microbes in the intestine produced toxic substances that were damaging to the nervous and vascular systems and caused humans to age. He had observed that Bulgarian peasants consumed large quantities of yogurt and had a long life expectancy. He also observed that natural fermentation of food by lactic acid-producing bacteria prevented the growth of putrefactive organisms. In his book, titled ‘The Prolongation of Life’, he concludes that: “as lactic fermentation serves so well to arrest putrefaction in general, why should it not be used for the same purpose within the digestive tube?” (1). Although Mechnikov’s concept of aging by “intestinal auto-intoxication” has no scientific basis today, Mechnikov’s theories remain influential and have contributed to the commonly held opinion that Lactobacilli display important functional characteristics that contribute to gut health.
Lactobacillus is a genus of rod-shaped, gram-positive, non-spore-forming, facultative anaerobic bacteria of the phylum ‘Firmicutes’ (2, 3). Lactobacilli metabolise carbohydrates to produce lactic acid making them the largest genus within the lactic acid bacteria (LAB) group. As of March 2020 the 261 species of the Lactobacillacae were reclassified into 25 genera (including 23 novel genera) due to their extremely high genotypic, phenotypic and ecological diversity (4). For the purpose of this review, ‘Lactobacillus’ will refer to those species previously classified as Lactobacillus. Traditionally, Lactobacillus species may be divided into three groups based on their metabolism. The obligate homofermentative group which ferment carbohydrates to produce lactic acid as the main by-product (e.g. L. acidophilus and L. salivarius), the facultatively heterofermentative group which, under certain conditions or with certain substrates, ferment carbohydrates to produce lactic acid, ethanol/acetic acid and carbon dioxide as by-products (e.g. L. casei and L. plantarum) and the obligately heterofermentative group which always ferment carbohydrates to produce lactic acid, ethanol/acetic acid and carbon dioxide as by-products (e.g. L. reuteri and L. fermentum) (5).
Lactobacilli have colonised multiple areas of the human body, most notably the digestive tract including the oral cavity, and the female genital tract (6). The association between Lactobacilli and humans is a mutualistic relationship, with Lactobacillus species offering the host aid in digestion of certain dietary substrates, as well as protection from pathogens, in return for accommodation and nutrients (7). Lactobacillus species possess qualities that are commercially desirable both as health supplements and as tools in the food technology sector. The main uses for Lactobacilli are in the manufacturing process of fermented dairy, meat, or vegetable foods and sourdough breads, and they are also widely used as probiotics i.e., live micro-organisms that, when administered in adequate amounts, confer a health benefit on the host (8, 9). Lactobacilli have been granted a ‘generally recognised as safe’ (GRAS) status from the U.S. Food and Drug Administration (USFDA) and ‘qualified presumption of safety’ (QPS) status from the European Food Safety Authority (EFSA) thus making their use in food manufacture relatively straightforward. Due to their economic importance, Lactobacilli are highly studied and, relative to other bacterial genus’, are well characterised in terms of genomics and also their interactions with humans in terms of both health and disease. These features make Lactobacillus species ideal probiotic candidates.
Considering the widespread media attention that the microbiota have attracted in recent years with many news outlets covering this link between microbes and health it is little wonder that the commercial probiotic market is worth approximately $54 billion USD worldwide (10). For a list including some of the most common Lactobacillus strains found in probiotic products and their sources see George Kerry et al. (11). Although the strain L. rhamnosus GG is one of the most heavily studied, L. acidophilus is the most commonly used in commercial products. For an in-depth review of common commercial Lactobacillus strains see the chapter by Tang and Zhao in the book ‘Lactic Acid Bacteria: Omics and Functional Evaluation’ (12).
In 2002 a joint Food and Agriculture Organisation (FAO) and WHO working group released guidelines for the evaluation of probiotics in food (8). The minimum requirements include: assessment of strain identity (genus, species, strain), in vitro tests to show probiotic effects (e.g. resistance to gastric acidity, digestive enzymes and bile acid, and anti-microbial activity against pathogens), safety assessment to prove that the probiotic product is safe for consumption and without contamination, and finally in vivo studies to authenticate the purported health claims of the product (13). In Europe, the EFSA considers the terms ‘probiotic’, ‘prebiotic’ and the words ‘live’ or ‘active’ when used in relation to bacteria, to be health claims. Legislation on products purporting to carry health claims are strictly controlled although in recent years countries including Spain, Denmark and the Netherlands have released national guidelines allowing use of the word probiotic under certain conditions. This has renewed appeals to the EU Commission to reconsider the strict regulation. Unfortunately, in the US and Canada the FAO/WHO guidelines are not followed and indeed the use of the term probiotic has not been controlled by legislation. This means that any product can use the word ‘probiotic’ on its packaging thereby making it extremely difficult for consumers to determine which products are genuine probiotics that may actually be beneficial for their health (14).
In order to be considered efficacious, a probiotic must have the capacity to survive in the gastrointestinal (GI) tract, must resist the low pH of the stomach, must lack antibiotic resistance genes and must provide a clear benefit to the host (15). Of all probiotics, Lactobacillus species are the most widely used and studied (16). The main probiotic Lactobacillus species include: L. acidophilus, L. brevis, L. casei, L. delbrueckii subsp. bulgaricus, L. delbrueckii subsp. lactis, L. fermentum, L. gasseri, L. helveticus, L. johnsonii, L. paracasei subsp. paracasei, L. plantarum, L. reuteri and L. rhamnosus. There is much research into the potential health benefits of Lactobacillus species, although evidence indicates that many features of these probiotic bacteria are both species and strain -dependent (17). Despite this it has been observed that a single probiotic species may demonstrate improvement in different patient cohorts eg. L. rhamnosus GG (18) and additionally that a range of different probiotics or probiotic combinations may demonstrate efficacy in the same condition eg. C. difficile infection (19) highlighting the existence of conserved beneficial features. As is the case for many translational therapies, efficacy is not always maintained from in vitro observations through preclinical to clinical studies for a myriad of factors. Unfortunately, for many probiotics, one of these factors being that the mechanisms of action by which beneficial clinical outcomes are achieved have yet to be elucidated (20). The consequences for this mean that we are not utilising these tools to their full potential, opportunities for improving existing treatments may not be realised and we are at risk of probiotic treatments resulting in worse outcomes for particular subsets of patients (21). Additionally, mechanistic data may be required in order to gain approval from regulatory bodies for health claims – a mode of action is defined by the World Health Organisation (WHO) and EFSA as ‘a biologically plausible sequence of key events leading to an observed effect supported by robust experimental observations and mechanistic data’ (22). Kleerebezem and colleagues (23) propose the establishment of a translational pipeline connecting mechanistic insights to probiotic efficacy in order to improve the initial selection of probiotic strains by being able to predict their expected outcomes while supporting the design of the most appropriate clinical trials in well-defined subpopulations. They also suggest that this would be used in the inverse allowing us to predict explanations for observed clinical effects by drawing on existing knowledge of the probiotic modes of action. Determining the precise beneficial features of probiotics would certainly allow us to make better predictions for improved health outcomes.
On this note, further research is exploring ways to increase the efficiency, efficacy, safety and quality of probiotics by isolating probiotic-derived biomolecules. These have been described as postbiotics, paraprobiotics, heat-killed probiotics, Tyndallised probiotics among others: generally referring to metabolic products or secreted products of the bacteria, non-viable microbial cells (intact or broken) or crude cell extracts; specifically this includes enzymes, secreted peptides/proteins, bacteriocins, short chain fatty acids (SCFA), organic acids and cell envelope components of bacteria including peptidoglycans, teichoic acids, cell surface proteins and cell wall polysaccharides (24). The International Scientific Association for Probiotics and Prebiotics (ISAPP) has released a consensus statement on the definition of postbiotics establishing it as a “preparation of inanimate micro-organisms and/or their components that confers a health benefit on the host. Effective postbiotics must contain inactivated microbial cells or cell components, with or without metabolites, that contribute to observed health benefits”. (25). Postbiotics maintain several advantages over probiotics as described by Pique et al. (26): (I) No risk of translocation from the gut lumen to blood among vulnerable subjects, (II) No risk of acquisition and transfer of antibiotic resistance genes, (III) No risk of interference with normal gut colonisation in neonates, (IV) Release of active molecules from the disrupted inactivated cells, pass through the mucus layers and stimulate epithelial cells more directly, (V) Loss of viability by cell lysis can produce further more complex beneficial effects and (VI) Easier to extract, standardize, transport, and store. Accordingly, the use of postbiotics may very well represent a much-improved alternative to live probiotics and would be a likely replacement for them in future. A recent review has nicely summarised the composition and beneficial functions of postbiotics from Lactobacillus species (27). In short, postbiotics derived from Lactobacillus comprise a range of molecules which have various beneficial effects including immunomodulation, epithelial barrier protection, anti-pathogenic effects and anti-tumour effects.
Lactobacilli have demonstrated efficacy in treating various conditions including bacterial vaginosis, atopic dermatitis, and upper respiratory tract infections (28–30). However, as first proposed by Mechnikov over 100 years ago, the majority of Lactobacillus probiotics are consumed with a view to improving GI health. In the century since this hypothesis, interest and knowledge surrounding this subject has grown massively, however the potential for further growth in this area is exponential and much more work will be required before we fully understand and profit from the complexities of the relationships between Lactobacillus and gut health.
Lactobacillus spp. and Intestinal Barrier Integrity
The GI mucosa is the largest and one of the most critical barrier sites of the body where foreign antigens, microbes and potential pathogens come into close contact with the host’s immune system. It is a semi-permeable barrier which allows for the absorption of nutrients and immune sensing while restricting the influx of potentially harmful antigens or microbes. The GI barrier is composed of four major elements: the commensal microbiota, the mucus layer – which contains secretory IgA molecules (sIgA) and anti-microbial peptides, the intestinal epithelial cell (IEC) monolayer, and the gut associated lymphoid tissue (GALT) – which constitutes various populations of immune cells in compartments along the GI tract. The complexity of regulating this semi-permeable barrier is mitigated by dynamic inter-regulation between these elements which work together to maintain intestinal barrier integrity and homeostasis (31). Loss of intestinal barrier function has been implicated as an early event in the pathogenesis of various GI disorders, such as coeliac disease and inflammatory bowel disease, as well as systemic disorders including type I diabetes, obesity and multiple sclerosis (31).
Intestinal barrier function may be enhanced with the intake of non-pathogenic micro-organisms which augment the physical barrier of the mucus layer, enhance innate defence against pathogens and decrease paracellular permeability of IECs (32). Lactobacillus strains consumed as probiotics are thought to modulate the native intestinal microbiota and improve health via multiple mechanisms of action. As illustrated in Figure 1, probiotics strengthen intestinal barrier function by increasing mucus production, stimulating release of anti-microbial peptides, and production of secretory immunoglobulin A (sIgA) production, increasing tight junction integrity of IECs and providing a competitive resistance against pathogens such as for host colonisation receptors (33, 34).
Probiotic mechanisms of intestinal barrier enhancement.
Mucus Production
Goblet cells (GC) of the GI tract express rod-shaped mucins which either adhere to the epithelium or are released into the GI lumen. These mucins are highly glycosylated and link together via di-sulfide bonds to form a glycoprotein matrix that shields the intestinal epithelium from gut luminal contents (containing digestive enzymes), prevents interaction between pathogenic antigens/bacteria and the epithelial monolayer, and also aids GI motility. The mucus layer is generally between 50-800 µm thick and in healthy individuals the first 30 µm closest to the epithelial surface should be free of microbes. Lactobacillus species are believed to enhance intestinal barrier defence by promoting mucus secretion. In vitro studies have demonstrated that conditioned media from L. casei T21 can up-regulate the mucosal protective MUC2 gene in colonic epithelial cells (Caco2 and HT29) challenged with C. difficile (35). Although it has been proposed that acid may stimulate enteric cells to produce mucins (36) incubating HT29 cells with lactic acid did not replicate these findings indicating that other substances secreted by L. casei T21 are responsible for the increased gene expression. Similar results have also been obtained in the Caco-2 intestinal epithelial cell line using L. casei GG (37). In terms of in vivo studies, L. rhamnosus CNCM I-3690 has recently been shown to protect and/or restore the GC population and protect mucus layer thickness in mice following low-grade colon inflammation (38). Similarly, mice administered one of two strains of L. reuteri (L. reuteri R2LC or 4659) and exposed to DSS colitis displayed reduced colitis severity which the authors attribute at least partly to the increase in mucus thickness seen in mice given the probiotic both in control and inflammatory conditions (39).
The commercially available probiotic VSL#3 contains a combination of eight lactic acid producing bacteria of which four are Lactobacilli (L. plantarum, L. delbrueckii subsp. Bulgaricus, L. casei, L. acidophilus, Bifidobacterium breve, B. longum, B. infantis and Streptococcus salivarius subsp. thermophilus). Although the contribution of each bacterial strain cannot be clarified, both in vitro and in vivo experiments by Caballero-Franco et al. (40) using this probiotic in rats have indicated enhancement of the mucus layer measured by over-expression of mucin genes and increased basal luminal mucin content. Conversely, a similar study in mice failed to show altered mucin expression or mucus layer thickness using this probiotic (41). Further work is required to determine whether the in vitro effects of probiotics on mucus production are maintained in vivo.
Anti-Microbial Peptides/Factors
Host-produced GI anti-microbial peptides are generally categorised into cathelicidins and defensins. Cathelicidins are α-helical cationic peptides constitutively expressed in the GI tract which may also be activated by butyrate. Butyrate is produced by the enteric microbiota however few studies have examined the effect of probiotics on cathelicidin expression. Defensins are small, cationic peptides further classified into β-defensins, produced by epithelial cells throughout the intestine, and α-defensins, expressed in the small intestine. Defensins are constitutively expressed in the GI tract and display anti-microbial activity against many bacteria, fungi and some viruses. L. acidophilus PZ1138 and L. fermentum PZ1162, were shown to induce expression of human β-defensin-2 gene in Caco-2 cells via classic pro-inflammatory mechanisms (42). L. reuteri (FINELACT®) administered to broiler chicks was associated with anti-microbial peptide modulation in the cecum and ileum in addition to upregulation of pro-inflammatory mediators (43).
In addition to host-derived anti-microbial peptide stimulation, commensal bacteria also produce anti-microbial factors to aid in host barrier defence. These factors include short chain fatty acids (SCFA), hydrogen peroxide and bacteriocins. Lactobacilli alter luminal pH by producing lactic acid. This inhibits the growth of some bacteria and damages the outer cell membrane of Gram-negative bacteria, including E. coli O157:H7, Pseudomonas aeruginosa, and Salmonella enterica serovar Typhimurium making them more vulnerable to other anti-microbial molecules (44). Anti-microbial activity by L. johnsonii NCC533 has been associated with lactic acid and hydrogen peroxide production (45). Bacteriocins are small, ribosomally synthesised, heat-stable peptides produced by many species of bacteria which function to inhibit the growth of (bacteriostatic), or kill (bactericidal), other bacteria (46). Bacteriocins produced by Gram-positive bacteria generally exert their antibiotic effects by destabilisation of membrane function, typically against other Gram-positive bacteria, though some Gram-negative bacteria may also be susceptible (47). Lactobacillus strains produce SCFAs including acetate, propionate and butyrate, which have been shown to shown to increase transepithelial electrical resistance and stimulate the formation of tight junction in Caco-2 intestinal epithelial cells in vitro via inhibition of the NLRP3 inflammasome and autophagy (48). L. plantarum strains produce several bacteriocins which demonstrate anti-microbial activity against food borne pathogens such as Listeria monocytogenes as well as food spoilage bacteria are applied in food production to reduce the use of chemical preservatives (49). Corr et al. (50) demonstrated that Abp118 produced by L. salivarius UCC118 in vivo protects mice against L. monocytogenes infection. Two other bacteriocins analogous to Abp118 have since been identified by comparative genome hybridisation analysis from L. salivarius DPC6488: salivaricin L and T. Both bacteriocins demonstrated inhibitory activity towards L. delbrueckii subsp bulgaricus LMG 6901 with salivaricin L additionally inhibiting L. monocytogenes NCTC 11994 and L. innocua DPC3572 (51).
Secretory IgA
The production of IgA is an important strategy utilised by the GI tract to generate immune protection in a non-inflammatory mode (52). IgA dimers (secreted by intestinal B cells located in Peyer’s patches or lamina propria) interact with the polymeric IG receptor (pIgR) on the basolateral surface of epithelial cells, translocate to the surface of the epithelial cells and are released as sIgA (53). sIgA primarily promotes the maintenance of suitable commensal bacterial communities in the gut by binding dietary antigens and potential pathogens in the mucus and down-regulating the expression of pro-inflammatory bacterial epitopes on commensal bacteria (54). Furthermore, sIgA enhances the intestinal barrier by blocking microbial components involved in epithelial adherence, facilitating intraepithelial defence against pathogens and microbial products and enabling antigen sampling (55). In addition, locally released IgA dimers function to remove micro-organisms that have breached the epithelial barrier by facilitating their removal or promoting their clearance by binding to the CD89 receptor on immune cells such as dendritic cells, neutrophils and other phagocytes (56). Although commensal bacteria are believed to induce sIgA expression in the GI tract the mechanisms are not well understood, although there appear to be differences in the microbes responsible for small intestine and large intestine sIgA induction (57). Various Lactobacillus strains including L. paracasei MCC1849, L. gasseri SBT2055, and L. plantarum AYA are known to increase sIgA levels in the small intestine (58–60). In a clinical trial of children 12 to 24 months old, supplementation with L. plantarum IS-10506 increased sIgA faecal titres and a significant positive correlation was observed between this and TGF-β1/TNF-α ratios (61). The authors propose a probiotic induced immune activation of TGF-β1, which in turn increases the production of sIgA.
Epithelial Cell Barrier
As previously described, IECs form a monolayer of cells which act as a physical barrier between the external environment of the gut lumen and the host’s immune system. The integrity of this barrier is ensured by tight junctions (TJ) which are multi-protein complexes that bind the cells tightly together as well as adherens junctions, gap junctions and desmosomes. TJs are located towards the apical side of the epithelial cells. They consist of transmembrane proteins (claudin, occludin, and junctional adhesion molecules) which interact extra-cellularly with similar proteins of TJs in neighbouring cells and intra-cellularly with the cells own cytoskeleton via zonula occludens (ZO) proteins and filamentous actin (62). Loss of TJ integrity has been observed in chronic inflammatory disease, and mechanisms of disrupting TJ proteins in order to breach the GI barrier have been observed in infection by enteric pathogens such as C. difficile, E. coli, Salmonella Typhimurium, C. rodentium, Vibrio cholera among others (62). It has been demonstrated that L. rhamnosus GG ATCC 53103 up-regulates ZO-1, claudin and occludin expression in Caco-2 cells (63). This probiotic strain has been observed to increase levels of ZO-1 expression and enhance distribution of claudin-1 protein as a protective mechanism against enterohemorrhagic E. coli O157:H7 infection (64). Increased expression of ZO and occludin was also observed using various L. plantarum strains (L. plantarum WCSF1, CGMCC 1258, and MB 452) (65–67). L. plantarum WCSF1 administration into the duodenum of healthy human subjects increased ZO-1 and occludin staining in the vicinity of TJ structures via activation of TLR-2 (65). The addition of a TLR-2 agonist PCSK to Caco2 monolayers in vitro increased staining of occludin in TJ regions and was protective against epithelial barrier disruption. TLR-2 ligand binding leads to PKC activation which has been demonstrated to cause translocation of tight junction components (68) thereby it is likely that barrier integrity is enhanced by alterations to composition of tight junction proteins rather than an increase in these proteins. Lactobacillus species may also stabilise adherens junctions by increasing expression of E-cadherin, as well as by strengthening the E-cadherin/β-catenin complex (which connects adherens junctions to the cytoskeleton) via enhanced phosphorylation of β-catenin (69). In a clinical study of small intestine barrier function, biopsy samples demonstrated that L. plantarum strain TIFN101 and to a lesser extent L. plantarum WCFS1 and CIP104448, modulated an increase in gene expression of TJ and adherens junction proteins (70).
Competitive Resistance
Lactobacilli also aid intestinal barrier resistance to invading pathogens by competing for binding sites on IECs, glycoproteins in the mucus layer or to the plasminogen of extracellular matrix (71). In order to facilitate the necessary interactions with host cells, Lactobacillus species display various different components on their outer surface. These may include cell wall proteins, S-layer proteins, pili proteins, and moonlight proteins (72) (see Figure 2). These surface proteins facilitate adhesion of Lactobacilli to the host, for example LPXTG proteins found in several Lactobacillus strains are cell surface proteins covalently bound to the peptidoglycan layer and can bind to both mucus and epithelial cells (73). Several Lactobacillus strains possess a crystalline, glycoprotein surface layer, also known as the S-layer, non-covalently anchored to the peptidoglycan cell wall (74). The S-layer S-proteins of L. acidophilus ATCC 4356 have demonstrated anti-viral activity against alphavirus and flavivirus infection of 3T3 cells by blocking pathogen adhesion to C-type Leptin receptors (DC-SIGN) an attachment factor which strongly promoted viral infection (75). Further work is required to elucidate the mechanism for this, which may be multi-faceted, though the time-dependant aspect of the anti-viral function may indicate that S-layer proteins are activating downstream anti-viral signalling pathways.
Representation of the Lactobacillus cell surface structure including important effector molecules.
Pili are long protein structures, first observed in a non-pathogenic bacteria in L. rhamnosus GG, which protrude from the bacterial cell playing a major role in adhesion to the epithelium. In L. rhamnosus GG (ATCC 53103) SpaC pili have been demonstrated to out-compete the pathogenic Enterococcus faecium (76).
Moonlighting proteins are multifunctional proteins in which one polypeptide chain performs more than one unrelated biochemical or biophysical function (77). In Lactobacilli, moonlighting proteins may have a primary function as intracellular proteins but are also found on the cell surface where they facilitate adhesion, for example, L. plantarum 299v (78), L. acidophilus (79), L. reuteri ZJ617 (80), display GAPDH on their surface to mediate adhesion and colonisation of the GI tract. So far in the case of L. plantarum 299v it has been demonstrated that this results in competitive exclusion and displacement of pathogenic bacteria (81). The mechanism for the secretion of moonlighting proteins to the cell surface has not yet been elucidated.
L. rhamnosus R0011 and L. acidophilus R0052 adhere to Hep-2 and T84 intestinal cell lines in vitro preventing the binding of enterohemorrhagic E. coli and enteropathogenic E. coli (82). In Caco-2 cells, various strains of L. reuteri (LR5, LR6, LR9, LR11, LR19, LR20, LR26, and LR34) have been shown to adhere and inhibit and displace the binding of E. coli ATCC 25922, S. Typhi NCDC 113, L. monocytogenes ATCC 53135, and E. faecalis NCDC115 (83). It should be noted that competition for binding sites is species and strain -specific; L. rhamnosus ATCC 53103, L. gasseri DSM 20243, L. casei ATCC 393 and L. plantarum ATCC 14917 pre-treatments did not block enterohemorrhagic E. coli binding to human colon epithelial cell line C2BBe1 cells (although the L. rhamnosus strain prevented internalisation of E. coli into the cell line) (84). In a chronic stress model in vivo, pre-treatment with L. helveticus R0052 and L. rhamnosus R0011 reduced commensal adherence and translocation (85). Interestingly, in a hemorrhagic shock model in vivo, L. rhamnosus LMG P-22799 but not L. fermentum NumRes2 reduced bacterial translocation and cytoskeleton rearrangement despite both strains displaying similar pathogen exclusion properties in vitro in Caco2 cells (86). Indeed, L. fermentum NumRes2 increased bacterial translocation, primarily Lactobacillus spp., to the spleen highlighting the need for careful characterisation of the effects of individual.
Lactobacillus spp. and Gastrointestinal Infection
Understandably, the beneficial impact on gut health is one of the most widely studied topics in probiotic research. As discussed in the previous section, Lactobacilli protect the intestinal barrier from infection by promoting mucus production and barrier-related proteins, secreting anti-microbial substances such as SCFAs, bacteriocins and hydrogen peroxide which inhibit the growth of or kill pathogens, by modulating the host’s immune response to pathogens, and preventing adherence of pathogens and competing for binding sites. Thus, Lactobacilli are capable of preventing intestinal damage caused by certain bacterial infections. Lactobacillus probiotics have been demonstrated to inhibit the development of infection by pathogenic bacteria, such as C. difficile and C. perfringens (87), Campylobacter jejuni (88), S. Enteritidis (89), E. coli (90), Staphylococcus aureus (91), and Yersinia (92), among others. Two major GI disorders resulting from infection, H. pylori infection and antibiotic-associated diarrhoea, have been shown to greatly benefit from Lactobacillus probiotics and are outlined below.
H. pylori Infection and Lactobacilli
H. pylori infection is one of the most common bacterial infections in the world with more than half of the global population infected; though prevalence ranges from 24% in Oceania to 70% in Africa (93). H. pylori infects the epithelial lining of the stomach causing disorders such as peptic ulcer disease, chronic gastritis, and gastric cancer although many infected individuals are asymptomatic (94). Twenty percent of infected patients develop symptomatic gastritis, gastric or duodenal ulcers, gastric adenocarcinoma, or non-Hodgkin’s gastric lymphoma. The current recommended treatment for H. pylori infection involves multiple antibiotic drugs as well as a proton pump inhibitor however the effectiveness of this treatment is decreasing as H. pylori antibiotic resistance rises. The addition of a Lactobacillus probiotic (L. casei DN-114 001 (OAC-LC) and L. casei Shirota separately) and an L. acidophilus LB postbiotic have been shown to improve the efficacy of this therapy in various randomised controlled trials (95–97), however some trials have found no or only slight beneficial effects (98–101). Although the probiotic L. johnsonii NCC533 failed to eradicate H. pylori infection when administered alone, it did decrease inflammatory scores and urea breath test (used for the diagnosis of H. pylori infection) values (102, 103).
Cell-free spent culture supernatants (CFCS) derived from L. casei Shirota exhibited pH-dependant bactericidal activity against H. pylori in vitro (104). The CFCS of L. johnsonii NCC533 and L. acidophilus LB both resulted in the loss of H. pylori viability (105–107). Furthermore, the CFCS from these three Lactobacillus strains resulted in altered morphology of H. pylori bacteria to U-shaped or coccoid forms which are dormant forms of the bacteria with the coccoid form being less capable of colonising and inducing inflammation (108, 109). L. johnsonii NCC 533 and L. casei Shirota are also known to produce bacteriocins which are active against H. pylori (110). H. pylori is a spiral-shaped bacterium with multiple flagella allowing it to swim in the gastric mucus layer and interact with epithelial cells, an ability which is required for colonisation in the stomach (111). L. casei Shirota has been demonstrated to cause H. pylori to lose its flagellar motility due to transformation into dormant forms with no flagella and also by secretion of small anti-microbial compounds which inhibit swimming ability (104). Similarly, L. johnsonii NCC 533 also secretes compounds that inhibit the swimming ability of H. pylori (112). In order to survive in the low pH of the stomach, H. pylori expresses urease as a surface protein to neutralise the surrounding acidic environment. CFCSs from L. acidophilus LB and L. johnsonii La1 have been demonstrated to reduce urease activity of H. pylori (105, 106). In terms of adherence, L. acidophilus CFCS prevented the adhesion of H. pylori onto human HT-29 cells resulting in the death of adhering cells and reducing the urease activity of remaining adherent cells causing their lysis (105).
Antibiotic-Associated Diarrhoea and Lactobacilli
Antibiotic-associated diarrhoea (AAD) results from disruption of the normal microbiota of the gut by antibiotics with symptoms ranging from mild diarrhoea to more serious disease like pseudomembranous colitis (PMC) (113). AAD occurs in 5-30% of patients receiving antibiotics either during antibiotic therapy or up to 2 months after cessation of treatment. One of the major pathogens associated with AAD is C. difficile, responsible for 10-30% of normal AAD cases and 90-100% of severe cases such as PMC (114). Although other microbes including C. perfringens, S. aureus and Klebsiella oxytoca are associated with this disorder, they are not common (113). As the cause for AAD is known to be disruption of the normal intestinal microflora, and also due to the fears surrounding anti-microbial resistance, recent therapeutic research has focused on the use of probiotics or faecal microbiota transplantation to restore microbial equilibrium (115, 116). Though the mechanism of action of probiotics is not explicitly known in this case their efficacy seems to be maintenance of gut flora, out-competing pathogenic bacteria, preservation of intestinal barrier function and potentially immunomodulation. Treatment with several Lactobacillus strains including L. rhamnosus GG (ATCC 53103) and L. gasseri have been shown to be effective as a preventive measure for AAD (117). However, the effects are strain-dependent. A systematic review examined 51 randomised controlled trials and found that L. rhamnosus GG was significantly more effective than other probiotics, however L. casei species were most effective against C. difficile infection (118). Another recent review demonstrated similar results in children concluding that L. rhamnosus GG (ATCC 53103) can be safely given to prevent AAD and additionally to manage symptoms of acute gastroenteritis (119).
Lactobacillus spp. and Intestinal Inflammation
In humans, the immune system can be divided into the innate immune system and the adaptive immune system. Innate immunity is the first line of immune defence and is a non-specific response which acts as an immediate reaction to pathogens. Phagocytic cells such as natural killer (NK) cells, macrophages, monocytes and neutrophils recognise pathogenic targets and engulf and destroy them. Antigen presenting cells (APC) such as dendritic cells (DC) maybe activated via the innate response and in turn activate the adaptive immune response. The adaptive immune response relies largely on activation and differentiation of B and T cells. B cells recognise antigens via B cell receptors and act by secreting antibodies (humoral immunity). T cells recognise antigens via T cell receptors and differentiate into T helper cells (Th; CD4+) or cytotoxic T cells (CD8+). Th cells recognise antigen via MHC class I complexes and CD8+ cells do this via MHC class II complexes. Th cells differentiate into Th1 or Th2 effector cells which activate and regulate macrophages (Th1) and B cells (Th2) while CD8+ cells convert into cytotoxic T cells. In the GI tract the immune system is made up of the epithelial layer, the lamina propria and the gut associated lymphoid tissue. The GALT is populated by B and T cells as well as plasma cells, macrophages and M cells. APCs in Peyer’s patches take IgA antigen from epithelial cells to activate T cells and also transport it to lymphoid tissue of the lamina propria and mesenteric lymph nodes. M cells present in Peyer’s patches of the small intestine transport antigens, macromolecules, micro-organisms and inert peptides from the gut lumen into the tissue via adsorptive endocytosis. These antigens may then activate the innate and adaptive immune systems.
As alluded to in the previous sections, Lactobacilli play an immunological role within the GI tract of the host, strengthening the intestinal barrier and conferring protection from potential pathogens. Lactobacilli can interact with both the innate and adaptive immune response systems via micro-organism-associated molecular patterns (MAMPs) interacting with pattern recognition receptors such as Toll-like receptors (TLRs), nucleotide-binding oligomerization domain (NOD) receptors and C-type lectins expressed on immune cells or on tissues including intestinal epithelium (120). The Lactobacillus cell envelope comprises several types of molecules which act as MAMPs including the peptidoglycan multi-layer, teichoic acids (lipoteichoic acid (LTA) bound to the cell membrane and wall teichoic acid bound to the peptidoglycan layer), exopolysaccharides (EPS) along with cell surface adhesion molecules previously discussed (see Figure 2). The immunomodulatory effect of Lactobacilli is achieved with the release of cytokines, including interleukins (IL), tumour necrosis factors (TNF), interferons (IFN), transforming growth factor (TGF), and chemokines from immune cells (121). The inflammatory process depends on pro-inflammatory versus anti-inflammatory cytokines and in this way probiotics may act in an immunoregulatory or immunostimulatory manner. Immunoregulatory probiotics decrease inflammatory responses protecting the host against autoimmune diseases, inflammatory bowel disease and allergy and are characterised by IL-10 and regulatory T cell (Treg) production. IL-10 is an anti-inflammatory cytokine produced by monocytes, T cells, B cells, macrophages, NK cells and DCs to inhibit pro-inflammatory cytokines, chemokines and chemokine receptors protecting against intestinal inflammation. Immunostimulatory probiotics defend the host against infection and cancer development activating NK cells and developing Th1 cells via IL-12 production, and also defend the host against allergy by balancing Th1 and Th2 production. Mounting evidence would suggest that probiotic Lactobacilli have the potential to prevent or treat certain inflammatory conditions (122).
The activation of specific immune receptors by MAMPs on Lactobacillus species has been characterized to an extent. Peptidoglycan of L. casei Shirota, L. johnsonii JCM 2012 and L. plantarum ATCC 14917 has been shown to down-regulate IL-12 production via TLR2 (123). Peptidoglycan from L. rhamnosus CRL1505 demonstrated an enhancement of innate and adaptive immune responses ameliorating the Th2 response when administered nasally in mice (124). LTA of L. plantarum has been shown to elicit an anti-inflammatory response in both human and porcine intestinal epithelial cells via inhibition of IL-8 (125, 126). The knockout mutant for the SpaCBA pilus of L. rhamnosus GG demonstrated that not only are these pili essential for adhesion but also the knockout demonstrated an increase in IL-8 likely via LTA TLR2 signalling which suggests an immunomodulatory role for this adhesion molecule (127). The protective exopolysaccharide layer has also demonstrated immunomodulatory capabilities with EPS from L. rhamnosus RW-9595M inducing macrophage production of IL-10 and no induction of TNF-α, IL-6, or IL-12 (128) and L. plantarum 14 EPS decreasing the IL-6 and IL-8 production in response to an enterotoxigenic E. coli challenge in porcine epithelial cells (129). In mice, EPS derived from L. delbrueckii subsp.bulgaricus OLL1073R-1 fermented yogurt had an immunostimulatory effect, activating natural killer (NK) cells and inducing IFN-γ production in the spleen (130).
Some immunomodulatory effects are mediated by the metabolites of Lactobacillus, such as SCFAs, in particular, propionate, acetate, and butyrate. These postbiotics bind to specific receptors on intestinal epithelial cells to inhibit pro-inflammatory activity and Treg suppressive effects of neutrophils and macrophages (131–133). Indeed butyrate enemas have demonstrated efficacy and become an accepted treatment for diversion colitis though this is believed to be due to a relaxation effect on smooth muscle (134).Lactobacilli are also capable of producing antioxidants like glutathione (GSH) and can induce reductions in oxidative stress. Two strains of L. bulgaricus (L. delbrueckii subsp. bulgaricus B3 and A13) have been demonstrated to reduce lipid peroxidation, increase measurements of antioxidant enzymes, and reduce oxidative stress in a rat model of colitis (135). In a mouse model of gastric damage L. fermentum Suo significantly reduced malondialdehyde (MDA; a measure of oxidative damage) concentrations and serum concentrations of IL-6, IL-12, TNF-α, and IFN-γ (136). L. casei 114001 administered to rats increased the antioxidant capacity of plasma, liver and intestines and decreased MDA plasma concentration (137). In healthy human subjects, L. casei capsules administered with prebiotic inulin significantly decreased MDA and glutathione disulphide (GSSG; another measure of oxidation) concentrations and increased concentrations of antioxidant indicators: GSH, total GSH (GSHt) and free sulfhydryl group (-SH) in the plasma (138). Pre-treatment with L. acidophilus NCDC15 with inulin and L. rhamnosus GG MTCC 1408 with inulin in a model of colon cancer in mice lead to a reduction in MDA and an increase in antioxidants GSH-reductase, GSH-peroxidase and superoxide dismutase as well as fewer dysplastic changes (139).
Lactobacilli may also modulate the immune system by secretion of proteinaceous compounds. Proteins p40 and p75 released from L. rhamnosus GG ATCC 53103 both activated the Akt signalling pathway, inhibiting TNF-a –induced apoptosis in human and murine colonic epithelial cells and murine colon explants (140). Pre-treatment with L. rhamnosus GG milk prior to induction of dextran sulphate sodium –induced colitis in mice significantly reduced colonic inflammation and injury, suppressing cytokine-induced apoptosis and reducing H2O2-induced disruption of TJs. Depletion of two soluble proteins found in L. rhamnosus milk, p40 and p75, abolished these anti-inflammatory effects (141). L. rhamnosus GG ATCC 53103 increased production of the heat-shock proteins HSP25 and HSP72 in murine colon cells via secretion of soluble peptides which function via activation of MAPK signal transduction pathway (142).
There have been many reports of Lactobacilli influencing the immune system while also enhancing the intestinal barrier. In vitro, L. acidophilus PZ1138, L. fermentum PZ1162, and L. paracasei LMG P-17806 induced expression of human β-defensin-2 gene in Caco-2 cells via modulation of nuclear factor kB (NF-kB) and the activator protein 1 (AP-1) resulting in IL-8 expression (42). L. salivarius Ls33 peptidoglycan induced anti-inflammatory IL-10 production, and stimulated Treg responses via NOD2 rescuing symptoms in a tri-nitrobenzene sulfonic acid (TNBS) -induced colitis murine model (143). Enteral administration of L. rhamnosus GG decreased inflammation in the developing mouse colon, attenuating pro-inflammatory MIP-2 and TNF-α concentrations in an IL-10 receptor-dependent manner (144). In Caco-2 cells L. plantarum WCSF1 has been shown to enhance ZO-1 trafficking to TJ regions in a toll-like receptor (TLR)-2-dependent manner (65). In a porcine intestinal cell line, L. rhamnosus GG ATCC 7469 pre-treatment increased ZO-1 and occludin protein expression in a TLR-2-dependent mechanism and also attenuated enterotoxigenic E. coli –induced increases in TNF-α via a partly TLR-2-mediated mechanism (145).
Lactobacilli may interact with enterocytes, DCs, Th1, Th2 and Treg cells in their immunomodulatory capacity in the intestine. Studies in vitro and in vivo demonstrated that L. paracasei and L. acidophilus strains induced early innate and adaptive immune responses in developing mice and rats in terms of phagocytosis, polymorphonuclear cell recruitment and TNF-α, IL-6, IL-10, IFN-γ production in a TLR-dependent mechanism (146). Homogenates prepared from several probiotics including L. rhamnosus GG ATCC53103, L. rhamnosus LC-705, L. acidophilus NCFB-Lb1748, and L. bulgaricus ATCC 11842 have demonstrated the ability to suppress peripheral blood mononuclear cell proliferation and L. acidophilus homogenates also down-regulated expression of IL-2 and IL-4 (147). In a mouse model of colitis where IL-10-deficient mice were infected with H. hepaticus, the combination of L. paracasei 1602 and L. reuteri 6798 reduced mucosal inflammatory cytokines TNF-α and IL-12 and also reduced intestinal inflammation (148). In an in vitro model, L. sakei LTH681 induced the inflammatory cytokines IL-1β, IL-8 and TNF-α in Caco-2 cells while L. johnsonii La1 failed to induce pro-inflammatory cytokines and instead induced production of anti-inflammatory TGF-β (149). Co-culture of ileal explants from patients with Crohn’s disease with L. casei DN-114001 and L. bulgaricus LB10 resulted in decreased TNF-α expression as well as decreased numbers of CD4+ T cells within the inflamed mucosa (150). CFCS from L. acidophilus ATCC 4356, L. casei ATCC 334, L. lactis ATCC 11454 and L. reuteri ATCC 55148 down-regulated IL-8 expression in human HT-29 cells and had differing strain-dependent efficacies in decreasing pro-inflammatory cytokines (IL-1β, IL-6, TNF-α) and in increasing anti-inflammatory IL-10 production in LPS-stimulated monocyte-derived macrophages (151).
Inflammatory bowel disease (IBD) is an example of an intestinal inflammatory disease which may be modulated by Lactobacilli probiotics. IBD is a chronic, relapsing and remitting disorder characterised by inflammation of the GI tract with two main classifications: Crohn’s disease and ulcerative colitis. Although the cause of IBD is unclear, dysbiosis of the GI microbiota is a feature of the disorder and it is believed probiotics may have a therapeutic benefit by restoring microbial balance and also by immunomodulation (152). Data from both in vitro and in vivo studies in animal models of colitis are extremely promising in terms of reducing inflammatory markers and decreasing colitis severity (153–155), however the same cannot be said for clinical trials of probiotics in IBD. Although it would appear that probiotics have beneficial effects in inducing remission and increasing remission times in UC (156) this has not yet been demonstrated for CD (157). A meta-analysis recently showed that L. rhamnosus GG displayed no beneficial effects in IBD patients, though VSL#3 (a combination of eight lactic acid bacteria strains – of which four are Lactobacilli) was better than placebo in terms of a higher remission rate and lower relapse rate (158). Similarly, another recent meta-analysis and systematic review concluded that a combination of Lactobacillus probiotics and prebiotics were effective in UC, although probiotics in general were not effective in CD (159). Further randomised, placebo controlled, clinical trials will be required to clarify the role of Lactobacilli in IBD and to elucidate the most beneficial strain, dose, and mode of administration.
Conclusion and Future Perspectives
There is increasing evidence to suggest that commercial and clinical use of probiotics is outpacing proven science. A recent study in healthy human subjects given probiotic supplements indicated that the colonisation of the GI tract featured person, region and strain -specific differences. In some individuals colonisation did not occur with the GI tract demonstrating colonisation resistance to the probiotics. The authors conclude that considering the transient, individualised effect of probiotics, the development of new personalised probiotic approaches is merited (160).
Despite the ever-increasing prevalence of probiotic use, there are also many limitations and unknowns (161–163). Data from research trials on efficacy of probiotics in the treatment and prevention of disease can often have conflicting results with similar studies pointing to opposing conclusions. These confusing data are somewhat to be expected and may be accounted for given the extremely complex nature of host – probiotic – microbiota interactions. One must allow for the unique individual differences in human microbiota composition, due to age, health, diet etc., which may affect the response to the intervention and may even account for adverse effects. Risks associated with probiotic use are generally concerned with the safety of vulnerable patient cohort such as the elderly or the immunocompromised. Thorough elucidation of mechanistic properties and host interactions will required in order to determine the probiotic strains and required intake levels required to achieve the desired health outcomes. It is also of note particularly for probiotic use in healthy individuals, and indeed for mechanisms requiring microbe-host interaction, that evidence indicates that probiotics are unlikely to be capable of maintaining colonisation in the host with any differences in microbiota composition being transient and dependent on continued probiotic intake. In terms of study design, it is often the case that mechanistic observations are founded in in vitro cell populations which cannot give the full picture of host and microbiota interactions. These are not always supported by in vivo observations in animal models which themselves may be flawed given incompatibilities or inconsistencies between human and animal microbiomes. On top of this the variety of available and potential new probiotics is vast and, as we have seen, beneficial effects can be species or strain specific and may require combination with other probiotics or prebiotics to be effective. Additionally, it is often the case that probiotic trials are initiated and funded by components of the probiotic industry who have commercial interests and may have a motive to downplay adverse effects. Although systematic reviews and meta-analyses of existing studies go some way in trying to overcome biased or underpowered research and allow for observation of overall trends, they are not themselves immune from the introduction of bias. Large, long-term, multicentre randomised controlled trials of probiotics chosen based on mechanistic information with specific beneficial outcomes for specific human cohorts in mind and involving collaborations with non-affiliated groups should be the aim to truly separate the good from the ineffective or bad.
It is clear that we have a long way to go in understanding all of the complexities of the microbiota and the effects of probiotic bacteria for health. Far more in-depth clinical testing will be required in order to substantiate the health claims of commercially available probiotic health supplements. Further elucidation of the modes of action of beneficial probiotics in clearly defined subsets of populations will hopefully allow us to make better predictions about efficacy, improve clinical trial design and enable improvement in development of probiotic health strategies. Expansion in the field of bacterial-derived products i.e. postbiotics signals a more precise, effective and safer future for the probiotic health market. In the interim, those looking to improve their overall health by enhancing their GI microbial complexity might find it more advantageous to focus on consuming a healthy varied diet of grains, fruit, vegetables and fermented foods such as miso, nattō, kimchi and sauerkraut.
Author Contributions
Writing—original draft preparation, ED; writing—review and editing, ED and SC; Conceptualization, ED and SC; Funding acquisition, SC. All authors have read and agreed to the published version of the manuscript.
Funding
Funding for the Corr Lab is provided by Science Foundation Ireland [grant 19/FFP/6499].
Conflict of Interest
The authors declare that the research was conducted in the absence of any commercial or financial relationships that could be construed as a potential conflict of interest.
Publisher’s Note
All claims expressed in this article are solely those of the authors and do not necessarily represent those of their affiliated organizations, or those of the publisher, the editors and the reviewers. Any product that may be evaluated in this article, or claim that may be made by its manufacturer, is not guaranteed or endorsed by the publisher.
References
The Functional Roles of Lactobacillus acidophilus in Different Physiological and Pathological Processes
Abstract
Probiotics are live microorganisms that can be consumed by humans in amounts sufficient to offer health-promoting effects. Owing to their various biological functions, probiotics are widely used in biological engineering, industry and agriculture, food safety, and the life and health fields. Lactobacillus acidophilus (L. acidophilus), an important human intestinal probiotic, was originally isolated from the human gastrointestinal tract and its functions have been widely studied ever since it was named in 1900. L. acidophilus has been found to play important roles in many aspects of human health. Due to its good resistance against acid and bile salts, it has broad application prospects in functional, edible probiotic preparations. In this review, we explore the basic characteristics and biological functions of L. acidophilus based on the research progress made thus far worldwide. Various problems to be solved regarding the applications of probiotic products and their future development are also discussed.
Introduction
The Food and Agriculture Association (FAO) and World Health Organization (WHO) have highlighted that probiotics are live strains of microorganisms that have been carefully selected. When administered in sufficient amounts, probiotics can bring health benefits to the host [1]. There are a large number of probiotic bacteria that colonize the human intestine, which can also interact and co-evolve with the human body. They help the host to digest and absorb nutrients in food, metabolize toxic waste products, and produce amino acids and short-chain fatty acids necessary for normal human activities. They can provide definite health effects such as improving the host microecological balance while exerting other beneficial effects on the intestinal tract [2].
Since the early 1990s, a plethora of “probiotic” health products have swept throughout the global market. In the meantime, “probiotics” have become a hot international research topic. Probiotics have been extensively studied in a variety of diseases and have been demonstrated to produce a range of potential health effects. The most studied species include lactobacilli, bifidobacteria, and yeasts [3]. Among them, L. acidophilus, an important intestinal probiotic in the lactic acid bacteria (LAB) family, has had a great deal of focus placed upon it in terms of research and development, especially as it is so closely linked to human health. As such, L. acidophilus is widely considered to have probiotic effects and is one of the most commonly recommended microorganisms for dietary use [4]. Compared with many other probiotics, L. acidophilus has better resistance to both acid and bile salt. These characteristics facilitate the survival and proliferation of L. acidophilus in the harsh environment of the gastrointestinal tract. Its ability to survive under these conditions provides further opportunities for its products to successfully function in the human body. When the total amount of L. acidophilus reaches a certain threshold value, health promotion can be achieved. L. acidophilus has multiple effects on the human body, including nutritional effects, regulation of intestinal flora balance, enhancement of immunity, age-delaying and anti-cancer effects, and support of cholesterol reduction [5, 6].
In this review, the basic characteristics of L. acidophilus are summarized, its biological functions in various diseases are discussed, and future research directions and applications in the form of probiotic products are explored. We hope to unveil the relationships between L. acidophilus and various life activities and disease development so as to provide a theoretical basis for later research and application direction.
Basic Features and Functional Mechanisms of L. acidophilus
L. acidophilus was initially isolated from infant feces in 1900 and was officially named Lactobacillus acidophilus. Subsequently, a series of biological characteristics and functions have been studied. L. acidophilus is subdivided into many strain types, including L. acidophilus LA-1, LA-5, NCFM, and ATCC4356, DDS-1 [7]. Different strains also confer differing probiotic properties and functions. Since a strain may have multiple names, it is easy to confuse the strains during the process of understanding them and when undertaking research. Fortunately, most of the work on L. acidophilus, especially the work relating to its probiotic effects, has been done on particular strains.
Basic Features of L. acidophilus
L. acidophilus, within the genus Lactobacillus in the family Lactobacillaceae, is a gram-positive bacillus that does not form spores. They present as slender rods with a circular end, measuring 2-10 μm long. Most L. acidophilus strains are microaerobic bacteria, which grow better in anaerobic environments, or in 5~10% CO2, rather than in aerobic environments. Their optimum culture temperature is generally 35~38°C, and they basically do not grow in temperatures below 20°C. L. acidophilus has poor heat resistance and its optimum pH is 5.5~6.0. The growth characteristics of different strains are also slightly different from each other. L. acidophilus is eosinophilic and has good resistance toward acids and bile [8, 9]. It can grow and reproduce in environments where other LAB cannot grow. It can also use glucose, fructose, lactose and sucrose to carry out homotype fermentation and can produce DL-lactic acid via fermentation [10].
L. acidophilus is a species of beneficial microbial flora and has been proven to have many good probiotic characteristics that can be roughly divided into two categories. The first category covers the essential probiotic properties of L. acidophilus which have been demonstrated in vitro and include tolerance to low pH, bile resistance, adhesion to human colon cells in cell culture, antibiotic production, lactase activity, and product stability [11–17]. The second category includes the overall probiotic effects that have been observed in animal-level feeding studies, such as regulation of host immune responses, reduction of host serum cholesterol, improvement of host lactose metabolism, and prevention or treatment of infection [18–20]. Here, we summarize the basic probiotic properties and main biological functions of L. acidophilus (Fig. 1).
Probiotic properties and biological functions of Lactobacillus acidophilus.L. acidophilus is a species of beneficial microbial flora and has been proven to play an important role in many pathological and physiological processes. It has been shown to improve CVD and lactose intolerance, prevent and treat cancer, regulate immunity, and improve gastrointestinal diseases.
Functional Mechanisms of L. acidophilus
According to current studies, L. acidophilus participates in the host intestinal tract mainly through the production of metabolites and regulation of intestinal microbiota [21, 22].
First, L. acidophilus can regulate the balance of intestinal flora by reducing the intestinal pH and producing metabolites [21, 23]. The optimal pH of many intestinal pathogenic bacteria is neutral or slightly alkaline. Lactic acid produced by L. acidophilus metabolism can reduce pH, thus inhibiting the growth and reproduction of pathogenic bacteria [23]. In addition, some pathogenic microorganisms produce enzymes that can catalyze the conversion of carcinogenic precursors to carcinogens, such as azo reductase, nitro reductase, and β-glucosidase [24, 25]. L. acidophilus can not only inhibit the growth of these pathogenic microorganisms and reduce the production of these enzymes, but can also inhibit enzyme activity [25, 26]. Second, competition for adhesion sites with pathogenic bacteria is an important mechanism for L. acidophilus to inhibit the function of pathogenic bacteria, thereby interfering with their invasion into cells [27]. Surface the S-layer protein, extracellular polysaccharide and lipoteichoic acid of many strains can compete for adhesion with pathogenic bacteria [21, 28]. Third, the role of L. acidophilus in many diseases also depends on its ability to reduce serum cholesterol level [29, 30]. L. acidophilus can absorb and assimilate cholesterol [31, 32].
Although some mechanisms have been found, neither these mechanisms nor the influencing factors of L. acidophilus in the host have been fully studied and efforts should be made to explore them further.
Biological Functions of L. acidophilus
Risk Reduction of Cardiovascular Disease
Cardiovascular disease (CVD) is the leading cause of morbidity and mortality worldwide, accounting for about one third of global deaths [33]. The occurrence of CVD is related to many factors [34–36]. Increasing blood cholesterol is one risk factor that directly impacts CVD. In the 1970s, Mann and Shaper found that populations of particular African tribes generally possessed lower incidences of high serum cholesterol [37]. After investigation, they found that the residents of these tribes regularly drank yogurt fermented by L. acidophilus, suggesting that this bacteria might regulate blood lipids [37]. Since then, more researchers have paid attention to the uses of L. acidophilus and other probiotics relevant to cholesterol reduction and the cholesterol-lowering effects of L. acidophilus have been confirmed. In addition, studies have investigated the effects of L. acidophilus intervention on cholesterol reduction and atherosclerosis development in animal models.
Harrison and Peat reported that the addition of L. acidophilus to baby feed reduced infant serum cholesterol from 147 mg/100 ml on the 5th day down to 119 mg/100 ml by the 8th day of intervention [38]. This decrease in serum cholesterol levels was accompanied by a significant increase in the number of LAB. The number of Escherichia coli present in fecal samples also decreased [38]. Stepankova et al. found that supplementation of L. acidophilus ATCC 4356 also promoted the proliferation of bifidobacteria [39]. Gilliland and Walker showed that L. acidophilus NCFM was able to remove cholesterol from laboratory growth media [40, 41]. NCFM has been reported to ingest cholesterol in the presence of bile and in the absence of oxygen, both of which occur in the gut. The researchers tested these effects on young pigs and the results showed that feeding L. acidophilus to pigs significantly inhibited the increased serum cholesterol levels that are usually observed in individuals fed a high-cholesterol diet. Park and colleagues have reported that adding L. acidophilus 43121 to a high-cholesterol diet given to rats resulted in reduced serum cholesterol [42, 43]. Song et al. showed that L. acidophilus NS1 can reduce plasma LDL-C by increasing the expression of LDLR and SREBP2 in the liver [44].
Huang et al. found that L. acidophilus ATCC 4356 had a significant cholesterol-lowering effect on rats fed with a high-cholesterol diet by inhibiting the expression of NPC1L1 in the small intestine [31, 45]. Chen and colleagues found that L. acidophilus ATCC 4356 alleviated atherosclerotic lesions in ApoE−/− mice[46]. L. acidophilus ATCC 4356 inhibited oxidative stress by regulating the production of MDA, oxLDL and SOD, suppressed inflammation via regulations of TNF-α and IL-10 levels, and improved intestinal flora, resulting in blocked progression of atherosclerosis. However, it did not significantly reduce cholesterol levels. In different experimental models, many studies have produced different results due to inconsistent experimental methods. Therefore, the role and functional mechanism of L. acidophilus in reducing cholesterol and alleviating atherosclerosis require further detailed exploration.
Improvement of Gastrointestinal Disease Outcomes
Studies have shown that probiotics can regulate intestinal flora, play a beneficial role in inflammatory diseases such as ulcerative colitis (UC), and have been used effectively to treat and suppress human intestinal infections. Lightfoot and colleagues described the role of L. acidophilus NCFM surface layer protein A as a key effector in the prevention of colitis in mice [47] . Chandhni et al. also showed that the surface proteins in NCFM strains could reverse histopathological damage caused by colitis [48], thus providing a potentially safer option for the treatment of inflammatory bowel disease.
In the normal intestinal flora in humans, L. acidophilus plays a key role in inhibiting the growth of pathogens such as Salmonella enteritidis, Staphylococcus aureus, and Shigella dysenteriae. Therefore, studies have shown that L. acidophilus exhibited strong anti-inflammatory activities [49]. Moshiri et al. reported that L. acidophilus PTCC 1643 could affect the expression of TLR2 and TLR4 in HT29 intestinal epithelial cells under the action of Salmonella enterica serovar Enteritidis (SesE), and inhibit the inflammatory response caused by SesE infection [50]. Small intestinal bacterial overgrowth (SIBO) refers to the changes in the number or types of flora in the small intestine. It is considered to be a condition that can exist for many years without causing obvious symptoms although it is related to chronic digestive problems. Studies by Simenhoff and colleagues have shown that NCFM can inhibit the overgrowth of small intestinal bacteria, reduce the levels of toxic metabolites such as dimethylamine and nitrosodimethylamine in the blood, and positively affect intestinal colonization [51, 52], thus improving the nutritional status of patients. These observations support the use of L. acidophilus for the prevention and treatment of intestinal diseases. Further in vivo and in vitro studies are needed to elucidate the detailed mechanisms of these anti-inflammatory effects.
Improvement of Lactose Intolerance
Lactose intolerance, also known as lactose indigestion or lactose malabsorption, refers to the state in which the human body does not produce the enzyme lactase. After consuming milk or dairy products, some people might have diarrhea and other symptoms of intestinal discomfort due to the osmotic effect of the undecomposed lactose.
Previously, many studies have proved that LAB have the ability to be a source of lactase in the small intestine, which helps people with lactase deficiency to digest lactose. Related fermented dairy products may also enhance lactose tolerance [53].
Some probiotic studies have shown that L. acidophilus can improve lactose digestion or symptoms in lactose-intolerant patients [54, 55]. Among these studies, in vitro evaluation of the lactase levels of various probiotics has shown that the lactase levels of L. acidophilus NCFM were high when compared to all of the probiotics tested. Multiple studies have also shown that NCFM can improve lactose digestion and relieve symptoms of lactose intolerance such as bloating and diarrhea [56, 57]. A study speculated that the bacteria might metabolize lactose during digestion and transport it through the gastrointestinal tract. The study by Pakdaman et al. found that L. acidophilus DDS-1, a unique and edible strain, can improve lactose intolerance symptoms such as diarrhea, cramps, and vomiting [58]. However, a number of studies have shown the opposite effects. For example, Newcomer et al. demonstrated that dairy products containing L. acidophilus NCFM did not significantly improve human lactose intolerance [59–61]. The reason for these contradictory results has been associated with the levels of NCFM of L. acidophilus. Therefore, in order to better apply the functional properties of L. acidophilus and to improve lactose intolerance, it is essential to explore and adjust the probiotics levels and the formula with each product.
Prevention and Treatment of Cancer
Probiotics are considered a safe and cost-effective way to prevent or treat a variety of cancers, including colon and liver cancer. Several studies have suggested that consumption of cultured dairy products may reduce colon cancer risk, since the effects of diet are mediated by metabolic effects of intestinal organisms. The activities of β-glucuronase, nitroreductase, azoreductase and other microbial enzymes have been used to monitor colon cancer changes. Goldin and Gorbach observed that adding live L. acidophilus into the diet of carnivorous rats significantly reduced azoreductase, nitroreductase and glucuronidase activity [24, 25]. The incidence of colon cancer in rats with L. acidophilus NCFM was also lower. Their later study found that NCFM alongside antibiotics inhibited the growth of colon tumors in rats. In human, daily consumption of milk containing NCFM reduced the activity of these three fecal enzymes by a factor of two- to four-fold and reduced the incidence of colon cancer [24, 25]. In addition, they found that nitroreductase activity continued to decrease even three weeks after fermented milk intake was stopped, thus indicating a long-term change in colonic flora [24, 25].
Studies have shown that the extracellular polysaccharides (EPSs) synthesized by L. acidophilus have exerted health benefits by stimulating the immune response and fighting tumor cells [62]. The anticancer and immunomodulatory activities of EPSs synthesized by L. acidophilus have been proven to combat colon cancer and inflammatory liver cancer. Khedr and colleagues used male rats as a model and confirmed that L. acidophilus ATCC 4356 EPSs had immunomodulatory effects on liver cancer induced by diethylnitrosamine (DEN) and gamma radiation (IR) [63]. They proposed that L. acidophilus ATCC 4356 EPSs might be used as a safe and effective probiotic to prevent and treat liver cancer.
Regulation of Immune Capacity
The immune function of L. acidophilus is mainly conducted via regulating the body’s immune system, limiting pathogen colonization within the body, and controlling metabolic disorders and enteritis. Probiotics are used clinically to treat diseases caused by immune system disorders. This can significantly reduce infection time and respiratory tract infection frequency and also improve the therapeutic effects for allergic asthma.
The role of L. acidophilus in regulating the body’s ability to respond to immune responses has been demonstrated in previous studies. Wagner et al. confirmed that NCFM induced antibody- and cell-mediated responses to Candida albicans in immunodeficient mice [64]. The serum levels of IgG, IgA, and IgM were higher in euthymic immunocompromised mice and were thought to reduce the severity of candidiasis. Yoghurt prepared with yoghurt cultures containing NCFM, Streptococcus thermophilus, Lactobacillus bulgaricus and Bifidobacterium were tested for their effects on mucosal and systemic IgA and IgG responses in mice immunized orally with cholera toxin. The results showed that IgA against the cholera toxin was higher in the intestine and serum of the mice fed the formulated yogurt than that observed in the mice fed skim milk [65]. These results suggest that coculture including NCFM may increase immune responses to oral antigens.
Other Functions
We summarized the biological functions, processes, and effects of L. acidophilus– related strains in pathological and physiological processes (Table 1). However, the biological functions of L. acidophilus are far more extensive than what we have mentioned. The role of L. acidophilus in many other diseases is constantly being explored and new discoveries are being made. Studies have shown that kidney tissue damage can be alleviated by reducing oxidative stress, inflammation, and cell death [66]. Zhang et al. first explored the relationship between ATCC 4356 and renal ischemia-reperfusion injury (IRI) [67]. They found that L. acidophilus ATCC 4356 alleviated renal IRI through antioxidant stress and anti-inflammatory responses and improved intestinal microbial distribution in renal IRI mice. In the following treatment with ATCC 4356, the levels of anti-inflammatory factors (IL-4 and IL10) were upregulated, whereas the levels of pro-inflammatory factors (IL-1β, IL-8, TNF-α and IFN-γ) were downregulated. In addition, renal tissue apoptosis in IRI mice was reduced [67].
Table 1
The main biological effects of related strains of L. acidophilus.
Type of strain | Function | Major biological processes and their effects | Reference |
---|---|---|---|
L. acidophilus ATCC 4356 | Inhibit CVD progression | Inhibit oxidative stress by modulating the productions of MDA, oxLDL and SOD; suppress inflammatory status by regulating TNF-α and IL-10 levels; inhibit NPC1L1 expression in the small intestine; improve intestinal microflora; inhibit the development of atherosclerosis. | [31, 40, 45, 46] |
L. acidophilus NCFM | Assimilate cholesterol and control cholesterol levels. | [40, 41] | |
L. acidophilus 43121 | Affect cholesterol metabolism and reduce blood cholesterol levels. | [40, 42, 43] | |
L. acidophilus NS1 | Reduce plasma LDL-C by increasing hepatic LDLR and SREBP2 expression. | [44] | |
L. acidophilus NCFM | Improve gastrointestinal diseases | Strain surface layer proteins play an important role; alleviate Tcell-induced colitis by significantly reducing the proinflammatory response; preserve microbiome composition and intestinal barrier function; reverse histopathological damage caused by colitis; reduce the level of toxic metabolites. | [47, 48, 51, 52] |
L. acidophilus PTCC 1643 | Modulate the expression of TLR2 and TLR4 in HT29 intestinal epithelial cells challenged with SesE; enhance anti-inflammatory effects. | [50] | |
L. acidophilus NCFM | Improve lactose intolerance | Strain has a higher level of lactase, which metabolizes lactose during digestion and transits through the gastrointestinal tract, thereby improving lactose digestion. | [56, 57] |
L. acidophilus DDS-1 | Assist in breaking down lactose; improve lactose intolerance symptoms such as diarrhea, cramps and vomiting. | [58] | |
L. acidophilus ATCC 4356 | Prevent and treat colon cancer, liver cancer and other cancers | The exopolysaccharides of the strain have immunomodulatory and antitumor activities; regulate the TLR2/STAT-3/P38-MAPK pathway associated with inflammation against HCC. | [63] |
L. acidophilus NCFM | Stimulate the immune response; reduce the activities of β-glucuronase, nitroreductase, azoreductase and other microbial enzymes; produce compounds that inhibit tumor proliferation; reduce the incidence of colon cancer and inhibit the growth of colon tumors. | [24, 25] | |
L. acidophilus NCFM | Regulate immune capacity | Reduce levels of pro-inflammatory cytokines significantly and mobilize a systemic immune response; limit pathogen colonization in the body, control metabolic disorders. | [47, 64, 65] |
L. acidophilus ATCC314 | Manage inflammatory disorders | Regulate the secretion of inflammatory cytokines; reduce oxidative stress. | [70] |
Studies have shown that oral L. acidophilus can improve heart function in mice with myocardial infarction. Sadeghzadeh and co-researchers found that L. acidophilus was able to improve the hemodynamic and histopathological indicators of the ISO-induced myocardial injury rat model [68], providing obvious myocardial protection. In the future, probiotic supplements may become a new option for patients with ischemic heart disease.
L. acidophilus has been shown to have potential applications in the prevention and control of genitourinary and vaginal infections. Reid et al. precultured L. acidophilus NCFM with urinary and vaginal epithelial cells from healthy women and subsequently exposed them to different urinary tract pathogens. Results showed that NCFM competitively excluded these pathogens and effectively prevented and suppressed urinary tract and vaginal infections [69].
Rheumatoid arthritis (RA) is a common inflammatory joint disease. It has been reported that the ingestion of L. acidophilus ATCC 314 exerted anti-inflammatory and potent antioxidant properties in a collagen-induced arthritis (CIA) rat model [70, 71]. This suggests that L. acidophilus is a promising treatment that should be tested further in RA patient preclinical trials.
Applications and Future Prospects of L. acidophilus
As people pay more and more attention to health issues, it is of great importance that different kinds of probiotics within food are able to play a healthy role. Of these probiotics, L. acidophilus is one of the most commonly used microorganisms, as it is thought to have various beneficial effects on human health. These advantageous effects include lowering blood cholesterol, improving gastrointestinal diseases, and reducing the risk of lactose intolerance and carcinogenicity [72, 73]. Research and development into L. acidophilus has received widespread attention. It is referred to as the third-generation yogurt starter strain. L. acidophilus has good acid and bile salt resistance and produces a variety of antibacterial substances during metabolism. Due to these attributes, L. acidophilus strains have broad application prospects as functional, edible bacteria.
Despite these favorable characteristics, through our analysis of studies on L. acidophilus which highlighted our present understanding of the current application status, we found that there are also many problems and limitations in the research and application of L. acidophilus. First, due to different naming methods, the same strain may have multiple names. This may lead to confusion during literature searches and research. Important findings could be missed, resulting in incomplete information collection. Second, due to the different research methods used to investigate probiotics, results from different research groups may deviate from each other. Different concentrations and ratios of probiotics have been shown to have different effects, therefore the health benefits of certain disease symptoms remain to be proven. Further research on the optimal strains, doses and dosing algorithms are of key importance for future research. In addition, different species of L. acidophilus can exhibit similar probiotic effects in vitro, but their properties differ significantly when evaluated in vivo.
Currently, probiotic regulation of intestinal flora is recognized as an interesting way to prevent certain diseases. Recent studies have proposed many mechanisms by which probiotics function, but the effectiveness of many probiotics has not been proven in different conditions, which has presently limited the promotion and application of probiotics. There are probably several main reasons why these research limitations occur, including too many low-quality studies, variability within the microbiome, and great diversity between the probiotic strains used. However, some studies have reported reasonable and encouraging results that support further research into probiotics. With this in mind, we should put more effort into overcoming the difficulties. First, because most studies have, so far, focused on animal studies or small human research groups, it is difficult to assess the possible health effects of these probiotics in the general population. Therefore, we need to conduct more extensive epidemiological evaluations which take into account the variability between patients. Due to the high cost of such interventions, it is necessary to characterize strains well to select the strains that are most effective for a particular application. Second, we should identify bacterial markers of the microbiome in related diseases in order to gain sufficient clinical trial capacity. Furthermore, new methods for analyzing the microbiome and its function will greatly facilitate the research when studying large numbers of samples. Probiotics could serve as a low-cost, low-risk alternative to antibiotic treatment in order to prevent infection. We believe that the development of probiotics will open up another impressive field of research. Further research in this area may provide exciting avenues for healthcare strategies, as well as creating more economic and social benefits.
Acknowledgments
This work was supported by the National Natural Science Foundation of China (Grant No. 91849209) and Shandong Provincial Natural Science Foundation, China (Grant No. ZR2020QH016).
Footnotes
Conflict of Interest
The authors have no financial conflicts of interest to declare.